AI Insurance Policy Analysis and Coverage Checker - Get Instant Insights from Your Policy Documents (Get started for free)
Structural Failures During the 1923 Kanto Earthquake Engineering Lessons from Japan's Deadliest Seismic Event
Structural Failures During the 1923 Kanto Earthquake Engineering Lessons from Japan's Deadliest Seismic Event - Ground Motion Analysis Reveals Previously Unknown Fault Line Behavior in 1923
Advanced analyses of ground motions from the 1923 Kanto earthquake have unexpectedly revealed previously unknown aspects of fault line behavior during that event. Researchers discovered that the fault ruptured in two distinct areas, or asperities, each experiencing significant slippage. This finding challenges earlier understandings of the earthquake's dynamics. These new insights have made it possible to create more accurate numerical simulations of ground motions across a wider range of frequencies, improving the potential for predicting future seismic activity in the region. The focus on long-period ground motions, in particular, has become crucial for engineers designing structures that can withstand future earthquakes. The 1923 Kanto earthquake, being the most recent major event in the Tokyo area, makes these new discoveries particularly important for enhancing seismic preparedness within the Kanto region and refining building codes. This detailed examination of past ground motions is crucial for informing future mitigation efforts.
The 1923 Kanto earthquake's aftermath has been meticulously examined using modern ground motion analysis techniques, leading to a surprising revelation: the earthquake's impact wasn't solely due to the well-known major fault lines. These studies suggest a more complex picture, indicating that previously unidentified or less understood fault lines played a crucial, and perhaps underappreciated, role in the severity of the event. This new understanding challenges the way we conceptualize ground shaking patterns in the region. The analysis revealed that the intensity of ground shaking varied significantly across the impacted areas, with certain zones experiencing stronger tremors than previously estimated by older models.
This research delves deeper, proposing that these previously overlooked fault lines aren't isolated entities but rather integral components of a complex fault network. This network interacts dynamically during earthquakes, potentially influencing the rupture process in ways that make accurate risk assessments more challenging. Researchers utilized advanced tools, including sophisticated seismographic data integration and historical triangulation, to identify these features. These previously unidentified or underestimated geological aspects were successfully brought into sharper focus, uncovering intricate fault interactions that contribute to earthquake behavior.
Interestingly, this new understanding is crucial for anticipating aftershocks. The study suggests that the characteristics of aftershocks might be influenced by the initial rupture dynamics across the interwoven fault system. This discovery impacts our comprehension of the earthquake cycle and calls for a more nuanced approach to understanding and predicting the post-earthquake seismic sequence. This research demonstrates how ground motion simulations can be improved to reflect the updated fault behavior. Consequently, a re-evaluation of engineering principles is essential. Newly designed structures, when informed by this knowledge of the interconnected fault network, could exhibit enhanced resilience during future seismic events.
Furthermore, this research prompts a reevaluation of building codes and design standards, specifically in regions with a history of powerful earthquakes. The data indicates that pre-1923 structures might not have considered these complex fault behaviors, thus highlighting potential weaknesses in older building designs. Incorporating the insights from the new understanding of the earthquake could significantly improve the safety and longevity of future construction. A vital outcome of this study emphasizes the need for multidimensional models that comprehensively capture the intricate interplay between faults. This more detailed understanding of how faults interact is critical for improved earthquake forecasting and more effective disaster preparedness strategies.
This reinterpretation of the Kanto earthquake serves as a powerful illustration of how historical events can be revisited with new perspectives and tools. It underscores the dynamic nature of geological processes, the need for constant refinement of our understanding, and the vital role of reassessing and potentially adjusting established engineering practices to ensure a higher degree of resilience to seismic hazards. These insights carry profound implications not just for future urban planning in the Kanto region, but for earthquake engineering practices on a global scale, reminding us that ongoing advancements in scientific knowledge necessitate the continuous refinement of seismic design and infrastructure strategies worldwide.
Structural Failures During the 1923 Kanto Earthquake Engineering Lessons from Japan's Deadliest Seismic Event - Wood Frame Building Collapse Patterns Led to Modern Timber Construction Standards
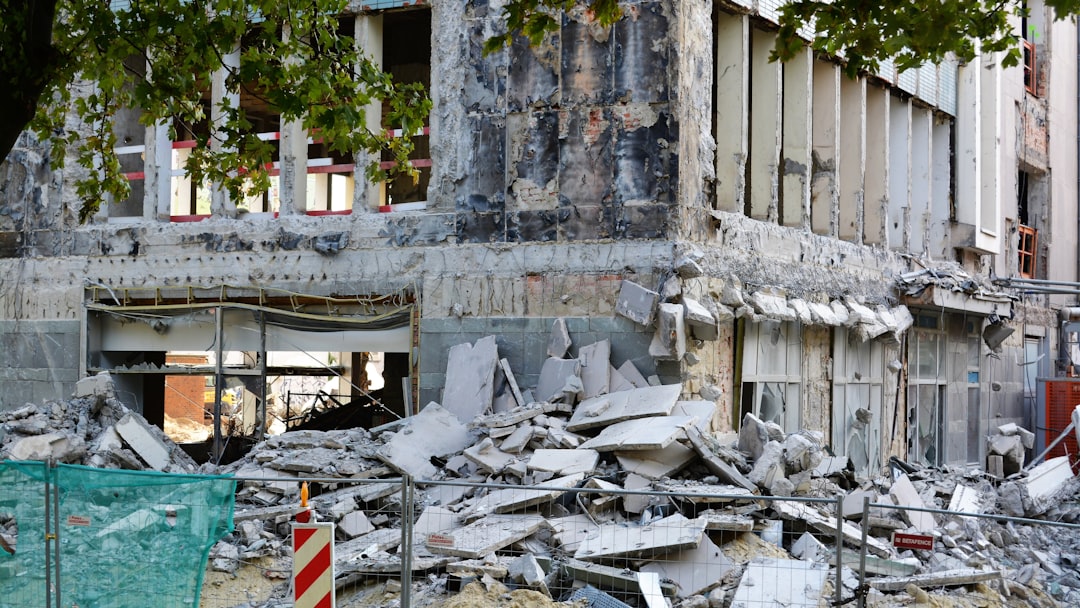
The 1923 Kanto earthquake exposed major flaws in the design and construction of wood-frame buildings, leading to widespread collapse. These failures revealed critical weaknesses in timber construction, particularly the tendency for timber to fail suddenly under tension, bending, or shear forces. Recognizing these patterns, engineers began to reexamine how wood structures are designed and built. The goal was to create stronger and more resilient structures that could withstand seismic events. The insights from the Kanto earthquake spurred the development of modern timber construction standards, focusing on improved structural connections and considering anticipated seismic loads during the design process. As timber structures grow in scale and height, it's becoming crucial to incorporate innovative construction techniques and rigorous analysis to ensure they can withstand increasingly demanding seismic forces. The need to prevent catastrophic collapse in taller timber buildings highlights the lasting impact of the lessons learned from the 1923 disaster. The insights gained from these failures have informed current timber construction practices, leading to more robust designs capable of withstanding future earthquakes, particularly in regions prone to seismic activity.
The widespread use of wood frame buildings in early 20th century Japan, based on the assumption that timber's inherent flexibility would be advantageous during earthquakes, was tragically proven wrong during the 1923 Kanto event. Many timber structures experienced significant failures, highlighting a critical oversight in design practices. The way these structures collapsed revealed that the configuration of vertical elements, such as wall and floor framing, played a surprisingly large role in overall building stability. This unexpected observation spurred a reassessment of timber framing techniques, shifting the focus from simply using wood to employing a more strategically designed system.
Subsequent revisions to building codes emphasized the need for braced frame systems, incorporating diagonal members to improve lateral stability. This was a substantial change in the way engineers approached timber construction, as it recognized the need to make timber structures stronger and more ductile under seismic loading. It became evident that a material's inherent properties alone were insufficient, and connection design between beams and columns needed special attention to avoid failure.
Further investigations into timber performance during the Kanto event unearthed a concerning pattern: poorly executed repairs and modifications to existing structures often increased their vulnerability during shaking. This emphasized the importance of following engineering standards when retrofitting buildings. Additionally, research into timber species has shown that certain types, like Japanese cypress, offer superior strength-to-weight ratios and hence potentially better seismic performance.
The Kanto earthquake prompted the development of rigorous timber testing methods to examine how various wood frame configurations react to dynamic loads. This empirical approach has formed the foundation for current timber construction standards. Observations of "pancake" collapses, where floors and walls failed sequentially, provided key insights for modern building designs, guiding engineers away from progressive collapse scenarios. We also learned that features like extensive overhangs contributed to increased problems during the quake. Contemporary codes now often restrict the use of such elements in seismic regions.
Since the 1923 disaster, the evolution of timber construction standards continues, a testament to the ongoing effort to learn from past structural failures. It's interesting to see that the relationship between intended building design and real-world performance during seismic events is still a major area of focus for the engineering community. The Kanto earthquake remains a stark reminder that the performance of a structure is complex and not simply a matter of the materials used. The subtle interaction of design intent and real-world performance in earthquakes is a vital element that engineers constantly seek to understand.
Structural Failures During the 1923 Kanto Earthquake Engineering Lessons from Japan's Deadliest Seismic Event - Fire Resistant Design Requirements Emerge After Secondary Conflagrations
Following the devastating 1923 Kanto earthquake, the widespread fires that erupted highlighted a critical vulnerability in building design: the lack of adequate fire resistance. The secondary conflagrations, fueled by debris and damaged infrastructure, significantly worsened the structural damage caused by the earthquake itself. Structures, including those made of concrete and steel, that appeared robust after the initial shaking proved remarkably susceptible to fire damage. The earthquake-induced weaknesses made them more vulnerable to fires compared to undamaged structures, emphasizing a crucial interaction between seismic and fire hazards.
This realization sparked a paradigm shift in design thinking, pushing engineers to move away from traditional, prescriptive methods of fire resistance. Instead, the focus turned to performance-based fire engineering, a more comprehensive approach that aims to quantify and manage the uncertainties inherent in fire scenarios. Current guidelines now reflect this shift, encouraging building professionals to think beyond simply meeting basic code requirements. The goal is to proactively assess a structure's performance under various fire conditions, factoring in the potential for increased vulnerability after an earthquake. This approach calls for the integration of fire safety into overall seismic design, a change that necessitates considering both hazards concurrently rather than as separate concerns. The lessons of the 1923 Kanto earthquake continue to shape our understanding of structural behavior in fire and earthquake scenarios, guiding modern practices towards safer and more resilient buildings.
Researchers have increasingly recognized the critical role of fire resistance in structural design following the 1923 Kanto earthquake. The widespread secondary fires, sparked by damaged infrastructure like gas lines and power grids, revealed a significant gap in pre-existing building codes. These fires disproportionately impacted wood-frame structures, leading to a dramatic shift in material preferences within urban areas. The near-total devastation of wooden buildings in Tokyo highlighted the need to incorporate fire safety as a core design principle alongside seismic resistance, a lesson that has reverberated through decades of subsequent building codes.
Engineers observed that using materials like brick and reinforced concrete significantly reduced fire damage, paving the way for a gradual transition away from traditional wood construction in earthquake-prone zones. The concept of compartmentalization, a design strategy aimed at containing fires within specific areas, emerged as a direct consequence of the observed fire spread during the Kanto earthquake. This led to a greater emphasis on designing structures capable of isolating fire damage to prevent catastrophic chain reactions and limit overall impact.
The Kanto disaster also stimulated research into passive fire protection techniques. The realization that structures needed to retain their integrity even under extreme heat conditions drove innovation in fireproofing coatings and barriers. Interestingly, the event even highlighted the influence of ventilation systems on fire behavior, leading to design considerations that aimed to minimize the movement of smoke and hot gases in case of a fire.
The secondary fires also prompted a reevaluation of emergency response and disaster planning. The lack of a coordinated approach between seismic and fire codes was deemed a significant contributor to the widespread damage. It became apparent that integrated design strategies were crucial for maximizing the safety of buildings exposed to both earthquake and fire hazards. This realization contributed to the development of new, more comprehensive design standards.
Sadly, many post-earthquake rebuilds did not fully incorporate these crucial lessons. Historical analysis shows that a significant portion of the Kanto earthquake’s structural losses were rebuilt without adequate fire resistance, suggesting a need for stricter code enforcement. This underscores the enduring importance of continually reinforcing the knowledge gained from past disasters to prevent future repetition. The interconnectedness of seismic and fire hazards remains a key consideration in modern building practices, with structures increasingly incorporating design elements that provide a higher degree of resilience against both dangers. This ongoing commitment to learning from historical events, particularly the devastating Kanto earthquake, remains crucial for achieving improved building safety and urban resilience.
Structural Failures During the 1923 Kanto Earthquake Engineering Lessons from Japan's Deadliest Seismic Event - Soil Liquefaction Impact on Foundation Systems Changes Engineering Practice
The 1923 Kanto earthquake starkly revealed the destructive potential of soil liquefaction on foundation systems, leading to widespread structural damage. Soil liquefaction, a phenomenon where saturated, loose soil loses strength and behaves like a liquid during strong shaking, can dramatically reduce the bearing capacity of the ground beneath structures. This loss of support can cause foundations to settle, tilt, or even fail completely, resulting in severe damage to buildings and infrastructure.
The devastation caused by liquefaction during the Kanto earthquake spurred significant shifts in engineering practices. Engineers and researchers started to seriously consider the susceptibility of different soil types to liquefaction, and they began incorporating methods into building design to mitigate the risks. This includes developing improved foundation systems, such as using deeper foundations or employing soil improvement techniques to increase the stability of the ground.
However, the science of understanding and mitigating liquefaction remains a work in progress. Modern geotechnical engineering now uses increasingly sophisticated computer models and innovative approaches like machine learning to predict soil liquefaction potential in different locations and under different seismic conditions. This knowledge, in turn, influences the design of foundation systems for new construction and helps determine the best way to retrofit older structures in areas at risk of liquefaction. The lessons learned from the Kanto earthquake continue to be a driving force behind this research, as engineers constantly strive to develop more reliable methods to protect against liquefaction-related damage in regions prone to earthquake activity. The aim is to ensure that future construction projects are designed with sufficient resilience to withstand the challenges posed by this dangerous natural phenomenon.
The 1923 Kanto earthquake starkly illustrated the destructive potential of soil liquefaction, a phenomenon where saturated, sandy soil loses strength and behaves like a liquid during seismic shaking. This event served as a critical turning point in how engineers approached foundation design, particularly in earthquake-prone zones. It became clear that understanding the soil conditions before construction was crucial, and that simply considering a site's general geological setting was insufficient.
Investigations after the Kanto earthquake revealed that liquefaction could occur at deeper soil levels than previously anticipated, a discovery that broadened our understanding of potential risk. This realization necessitated a more rigorous examination of soil profiles before designing foundations, especially those meant to support critical structures.
The earthquake spurred a shift towards more robust foundation systems like piles and caissons, which anchor structures deeper into stable soil layers, effectively reducing the impact of liquefaction. The goal was to find ways to establish a foundation that is less susceptible to failure during an earthquake. This practice, however, introduced its own engineering challenges.
The event also exposed the intricate interplay between the structure and the soil itself. It became apparent that foundation design should consider the potential dynamic behavior of the soil in addition to just bearing structural loads. Prior to the Kanto earthquake, the focus had been on static loads.
Seismic isolation systems, designed to decouple structures from ground shaking, faced challenges during the earthquake due to liquefaction. Engineers began to investigate hybrid approaches that combined isolation techniques with deep foundation methods to create more resilient structures. The idea of isolation from ground motion didn't quite perform as expected with all the ground instability.
The 1923 disaster pushed the revision of building codes, incorporating specific requirements to address liquefaction hazards. In some places, soil testing became a standard part of the engineering process, especially in regions known to be prone to earthquake activity.
Risk assessment models underwent revisions to better reflect the different soil types and moisture levels that affect liquefaction susceptibility. Engineers began to favor site-specific studies to improve the accuracy of predicting potential risks and develop more precise mitigation strategies.
The Kanto earthquake's lessons on liquefaction and its consequences have extended far beyond Japan's borders. Engineering practices in other parts of the world with similar geological conditions adopted these learned principles to reduce earthquake risks in their own territories. The idea that liquefaction needed to be addressed in building codes became widely accepted in earthquake zones.
Structures built before 1923 weren't designed with liquefaction in mind and retrofitting these older buildings to mitigate against liquefaction presents complex engineering challenges, especially due to their cost and complexity. The engineering needs are very specific to each building.
In the wake of the Kanto earthquake, researchers and engineers began to explore new materials and foundation techniques in hopes of developing more resilient systems that can absorb and disperse earthquake forces effectively. These advancements highlight the continuous effort to learn and improve upon the lessons learned in 1923.
It's remarkable how the Kanto earthquake's consequences have transformed our understanding of soil behavior and the design of foundation systems. The legacy of this event has been a global one, pushing us to continually enhance seismic design and preparedness. While much has been learned and applied, the challenges of designing structures in regions prone to liquefaction remain and will likely continue to be a major area of research and refinement for decades to come.
Structural Failures During the 1923 Kanto Earthquake Engineering Lessons from Japan's Deadliest Seismic Event - Bridge Infrastructure Failures Drive New Seismic Design Requirements
The 1923 Kanto earthquake exposed the weaknesses of existing bridge infrastructure, leading to significant failures. Many older bridges, especially those built before the 1970s, have shown susceptibility to seismic forces, highlighting the need for updated design standards. Engineers are increasingly focused on developing comprehensive lifecycle assessment frameworks for bridge infrastructure, considering not only the impact of earthquakes but also the effects of gradual deterioration and environmental damage, like corrosion. The varying levels of seismic risk across different areas require engineers to adapt their designs based on localized hazard assessments. While new strategies are being explored to improve the seismic resilience of aging steel bridge components, some traditional strengthening techniques have inadvertently led to increased localized seismic vibrations. Recognizing this, the focus has shifted towards more advanced Structural Health Monitoring methods and the implementation of probabilistic analyses for bridge systems, aiming to provide a better understanding of potential risk and inform decisions regarding design and maintenance for optimal earthquake resistance.
The 1923 Kanto earthquake exposed a significant gap in our understanding of seismic forces and their impact on infrastructure, particularly bridges. Prior to this event, bridge design often overlooked the dynamic forces generated during earthquakes, relying primarily on designs based on static loads. This oversight resulted in widespread bridge failures during the Kanto event, highlighting the need for a fundamental shift in engineering practices.
Many reinforced concrete bridges, built before the 1970s, suffered notably because they lacked design considerations for lateral forces that occur during earthquakes. Their designs did not ensure they could withstand these dynamic loads, which led to significant structural damage. In response, engineers started developing more sophisticated methods of assessing potential risks from these events, including incorporating lifecycle assessments for reinforced concrete bridges. This process specifically takes into account structural wear and tear plus the impact of corrosion caused by chloride contamination, issues that can accelerate the impact of earthquakes on aging bridge infrastructure.
Additionally, it became apparent that evaluating the reliability of bridge networks requires a more holistic view of hazards. There is a need to consider not just earthquake hazards, but how different hazards, including earthquakes, can combine or influence each other, particularly in regions prone to multiple events. Bridge systems simply aren't as isolated as they might seem during an earthquake.
The challenge of extending the lifespan of aging steel bridge piers exposed the limitations of many traditional strengthening methods, which sometimes inadvertently amplified local seismic vibrations, worsening the impact of the earthquake instead of improving them. It's clear there's still a lot of research needed in this area. In particular, seismic design standards differ significantly across regions, requiring customized approaches that factor in the specific seismic conditions of that location.
The Kanto earthquake also spurred advancements in Structural Health Monitoring (SHM) techniques. SHM has expanded beyond simpler evaluation methods to embrace much more detailed and complex evaluations. The goal is to gain a much better understanding of the current condition and the resilience of the bridge infrastructure under seismic loads.
More broadly, the earthquake also emphasized the increasing importance of considering the resilience of aging structures to earthquake hazards. This is a growing field of research that involves not just designing new structures, but assessing the risks associated with older ones and developing mitigation strategies for them. It's an area where the development of best practices is still a work in progress, making it challenging to fully evaluate the lifecycle risks of infrastructure.
The engineering community has also increasingly recognized that probabilistic analysis can be a valuable tool for better understanding bridge system resilience. The use of these methods allows engineers to make more informed design and maintenance decisions, particularly for older structures that have a complex history of repair and modification over time. It's become essential to better assess seismic risks that are inherent in bridge infrastructure.
The 1923 Kanto earthquake serves as a stark reminder of the importance of lessons learned from past events. Engineers are now far more sophisticated in their evaluation of earthquakes and the infrastructure damage they can cause. The lessons from the Kanto event continue to guide us as we strive to build safer and more resilient bridges that can withstand future seismic events.
Structural Failures During the 1923 Kanto Earthquake Engineering Lessons from Japan's Deadliest Seismic Event - Load Distribution Analysis from Collapsed Buildings Transforms Steel Frame Design
The 1923 Kanto earthquake's devastating impact on steel-framed buildings highlighted critical flaws in design approaches, leading to a major shift in how we analyze and design these structures. The event underscored the vital need to understand how seismic forces distribute loads within a steel frame, a previously underappreciated aspect of earthquake engineering. The collapse patterns observed in many buildings during the Kanto earthquake spurred the development of improved analysis methods. This includes simplified seismic sidesway collapse analyses and the use of sophisticated finite element modeling to predict the performance of steel frames during an earthquake.
Engineers are now recognizing that using accurate load distribution values, like those found in seismic design codes, are important. Although these values might sometimes be conservative, they are considered well-suited for evaluating how a steel frame will behave in a major earthquake. This emphasis on rigorous numerical analysis has led to a deeper understanding of how design loads impact structural integrity. There's also a growing awareness of the dangers of progressive collapse, which the Kanto earthquake tragically illustrated. This has resulted in a renewed focus on designing steel structures that are more resistant to failure, even under extreme conditions. Essentially, the 1923 disaster transformed the field of steel frame design by forcing us to be more cautious about load distribution and structural performance under earthquake conditions, shaping modern seismic design standards and emphasizing the need for better understanding of progressive collapse risks.
The 1923 Kanto earthquake exposed a surprising reality about how loads are distributed in steel frame buildings, particularly during seismic events. Researchers found that engineers had often misjudged the flow of forces through the structure, leading to unforeseen failure modes. This realization highlighted the complex interplay between structural components, where the behavior of the overall system, rather than individual elements, often dictated how a building would respond to ground shaking.
Early steel frame design often prioritized bending strength over shear resistance, but the earthquake demonstrated that shear failures could be equally destructive under dynamic conditions. Examining the collapsed steel structures revealed that different types of buckling correlated directly to how loads were distributed during the earthquake, pushing engineers to re-evaluate assumptions about lateral load impacts on frame components. It became evident that the connection points between different parts of the frame were not adequately designed for seismic forces. This finding emphasized the crucial role of these connection points in maintaining a building's integrity during earthquakes.
In response to these observations, Japanese building codes underwent a significant overhaul. These revisions integrated load distribution factors, considering both vertical and horizontal forces to achieve a more holistic approach to design. After the earthquake, more sophisticated analysis techniques became available. Using advanced modeling methods, engineers could better understand how different connection types impacted load distribution under dynamic conditions, influencing the development of modern design software capable of predicting structural response.
The lessons from the Kanto earthquake have inspired a more collaborative approach to steel frame design, merging insights from fields like seismic engineering, materials science, and structural dynamics. This cross-disciplinary approach has led to a deeper understanding of complex load paths and improved design practices. However, retrofitting existing buildings proved to be challenging, as modifications could introduce unforeseen load distribution issues. This highlighted the need for a deep understanding of the original design and its load-carrying capabilities when modifying existing structures.
The ramifications of the Kanto earthquake extended beyond Japan's borders. The insights gained about load distribution in steel frames during earthquakes influenced the global engineering community, particularly in regions with high seismic risks. These changes contributed to better design practices for steel buildings around the world, leading to enhanced building resilience in similar environments. This enduring impact underscores the importance of constantly learning from historical events to improve engineering practices and ultimately create safer, more resistant structures.
AI Insurance Policy Analysis and Coverage Checker - Get Instant Insights from Your Policy Documents (Get started for free)
More Posts from insuranceanalysispro.com: